Thank you for visiting nature.com. You are using a browser version with limited support for CSS. To obtain the best experience, we recommend you use a more up to date browser (or turn off compatibility mode in Internet Explorer). In the meantime, to ensure continued support, we are displaying the site without styles and JavaScript.
npj Clean Water volume 6, Article number: 64 (2023 ) Cite this article Invisible Fluorescent Uv Powder

The treatment of azo dye effluent from the dyeing process, as well as the sustainable development of the environment, can all be achieved through the use of green mycoremediation. In this in vitro study, Trichothecium roseum was isolated by the sedimentation technique from the environment of an unplasticized polyvinyl chloride pipe manufacturing plant and used to decolourize and optimise the biosorption percentage of Azo Lanasyn Navy M-DNL dye. T. roseum was also used to test the decolourization percentage of the dyes Lanasyn Navy M-DNL, Isolan Black 2S-LDN, and Isolan Yellow 2S-GLN. The outcomes showed that Lanasyn Navy M-DNL dye has the highest decolourization percentage (94%). Lanasyn Navy M-DNL dye was also found to be adsorbed onto the surface of T. roseum using scan electron microscopy and Fourier-transform infrared spectroscopy investigations. Under ideal optimisation parameters, the biosorption percentage of Lanasyn Navy M-DNL dye by beads of immobilised T. roseum cells was 96%, 97%, 96%, 97%, and 96%, respectively, for beads number 60, fresh weight 1 g, incubation temperature 25 °C, pH 6, dye concentrations 10 mg/l, and sucrose concentrations 10 g/l. Langmuir and Freundlich adsorption isotherms show good agreement between the Freundlich adsorption isotherm model and the adsorption process. Also, the elution cycle was found to be effective enough to be employed for five cycles using sixty beads of immobilised T. roseum cells. The current study suggests that T. roseum is an influencer of Lanasyn Navy M-DNL dye biosorption to create a successful green strategy for handling Lanasyn Navy M-DNL dye-contaminated effluents.
Abeer A. Ghoniem, Zeiad Moussa, … Ashraf Elsayed
Hossam EA Awad, Ahmad M. Mohammad & Emad A. Farahat
Sweat Parimita Beer & SK Tank
Azo compounds are widely employed in several industries, including the paper, carpet, and textile sectors. During the dyeing process, approximately 10–15 per cent of azo dyes are released into the water1,2. According to data, up until 1999, 50,000 metric tonnes of azo dyes were dumped into the environment, significantly polluting the ecosystem in the area3. According to estimates, the amount of dye in wastewater significantly increased in 2017 by 6.9 million tons4. When azo dyes are released into the environment, several negative consequences follow. Azole dyes have harmful effects on the aquatic ecosystem’s flora and fauna, as well as reduced photosynthesis and depleted dissolved oxygen5,6,7. Additionally, it has been demonstrated that the conversion of azo dyes into colourless aromatic amines causes cancer, is poisonous, and mutagenic8,9,10, while the presence of azo dyes in the freshwater system makes water taste bad. Physical and chemical methods have been developed to remove dyes. The techniques include membrane filtration, ion exchange, adsorption, chlorination, coagulation, and flocculation11, as well as sophisticated oxidation procedures (such as the application of hydrogen peroxide (H2O2), ozone (O3), and UV light). Evidence suggests that the majority of approaches employed, i.e., treating only specific types of dyes, are costly and time-consuming12,13,14,15. Further, from the perspective of the environment, these methods are found to be unsuitable as during the processes of dye removal, a high amount of sludge is generated (a secondary pollutant), and incomplete degradation of azo dyes occurs16; such as chlorination of Disperse Red 81 dye, which results in the formation of para-chloronitobenzine. The toxicity results of this newly formed compound were found to be more toxic than Disperse Red 81. Hence, chlorination has not been proven satisfactory in treating azo dyes17. Over the last few decades, research has confirmed that fungi have the unique quality of degrading dyes and removing several contaminants18. The fungal biomass demonstrated good adsorption capacity for Acid Blue 161 and Procion Red MX-5B19. The fungal hyphae exhibit biosorption phenomena, and the rough and porous topography of the fungal cell enhances the surface area for the binding of the toxic dye20. Biosorption, defined as the process of adsorption onto biological materials, both living and dead, has recently received increased attention for pollutant removal.
The various kinds of functional groups on the fungal cell wall, including carboxylic acids, carbonyl, hydroxyl, amides, and esters, enable effective interaction between the fungus and the surrounding pollution due to their vast range of functional groups. Fungi are good biosorbents that hold great potential for the removal of dyes because of their cost, capacity to be used repeatedly in industrial and food fermentation processes, and environmental friendliness21. Fungal cultures are utilised in decolourization procedures in still or agitated circumstances, either free or immobilised.
Fungal cells that have been immobilised have certain advantages over free cells, including improved decolourization efficiency, cell stability, and biomass reuse22,23,24.
Entrapment and attachment are the two main methods of cell immobilisation that are employed. For the entrapment technique, natural or synthetic polymers have been used for cell immobilisation. The microorganisms are entrapped in the agar, alginate, and chitosan25,26,27. Attachment or adsorption of microbial cells utilises the natural ability of cells to adhere to solid supports to form biofilms, which can exist as a single layer or be several millimetres thick28,29. In this study, the decolourization percentage of Lanasyn Navy M-DNL dye that is used for carpet dyeing was tested by DyStar Colours Distribution. The objective of this work was to isolate an original fungus for the decolourization of Lanasyn Navy M-DNL dye in aqueous solutions by employing immobilised cells of the filamentous fungus Trichothecium roseum with different parameters. Also, the biosorption mechanism was detected via SEM and FT-IR analyses. Moreover, the elution cycle was applied for Lanasyn Navy M-DNL dye biosorption using beads of immobilised T. roseum cells.
Under a 400-x magnification microscope, T. roseum was analysed. The fungal isolates were discovered to be whitish, powdery colonies reaching 90 mm in diameter on PDA media after seven days at 28 °C, as shown in Fig. 1a, fungal colony morphology on PDA plates, and Fig. 1b, c, fungal conidia and conidiophore under optical microscope examination. Conidia have smooth walls, are ellipsoidal to pyriform, and are septate. Conidiophore is upright and does not branch. Figure 1d shows a molecular identification of T. roseum based on a phylogenetic analysis of T. roseum AUMC15406, arrowed and aligned with closely related strains accessed from the Gen Bank. It showed 99.15–100% identity and 96–100% coverage with several strains of T. roseum. Other species of Trichothecium (Trichothecium ovalisporium and Trichothecium symposia) appeared in a separate clade in the phylogenetic tree.
T. roseum colonies 7 days growth at 28 °C on a PDA plate (a). T. roseum examination under an optical microscope revealed conidia and conidiophore (b, c). Scale bar = 10 µm. A phylogenetic tree showing the sequential relationship between the dyes decolourizing the fungal strain of T. roseum (d).
This experiment aimed to determine the highest decolourization percentage among the dyes using T. roseum. The data given in Fig. 2a shows that decolourization percentages of Lanasyn Navy M-DNL, Isolan Dark Blue 2S-GL, and Isolan Yellow 2S-GLN were 94%, 88%, and 85%, respectively, by T. roseum. So, Lanasyn Navy M-DNL was chosen to carry out the biosorption processes with beads of immobilised T. roseum cells. Also, results showed that the best decolourizing dye was Lanasyn Navy M-DNL.
T. roseum decolourization percentage Error bars are the standard error of the mean (a). Control of Lanasyn Navy M-DNL dye (b) and inoculated the Lanasyn Navy M-DNL dye flask after the incubation period (c).
These may be caused by variations in chemical composition, the quantity and arrangement of functional groups within the dye, the lengths of the dye organic chains, and the molecular sizes of the various dyes. Sandra et al. examined the adsorption of three azo dyes produced in industrial effluents by Cunninghamella elegans. They discovered that the adsorption removed 70% of reactive orange II, 85% of reactive black, 93% of reactive red, and 88% of a combination of them30.
SEM photomicrographs of Lanasyn Navy M-DNL dye and T. roseum at three different magnification powers (500×, 1000×, and 2000×) showed the morphological changes on the T. roseum surface after dye decolourization. T. roseum hyphae surface morphologies in solution medium at Lanasyn Navy M-DNL dye concentrations of zero and 10 mg/l are shown at low and high resolutions of SEM in Fig. 3. Hyphae surfaces at zero dye concentration are normal surfaces with no attached dye on the surfaces; I also noticed the formation of conidia (Fig. 3a–c). However, at 10 mg/l Lanasyn Navy M-DNL dye concentration, T. roseum surfaces with changes in hyphae surfaces were observed to have more dark-coloured hyphae due to the attachment of Lanasyn Navy M-DNL dye to hyphae surfaces and reduced conidia formation compared to zero dye concentration (Fig. 3d–f). Also, The SEM images of the T. roseum surface at zero concentration of Lanasyn Navy M-DNL dye and 10 mg/l concentration of dye revealed a difference in cellular morphology between zero and 10 mg/l concentration, indicating that the dyes were adsorbed onto the fungus hyphae.
The surface morphologies of T. roseum in solution at Lanasyn Navy M-DNL dye concentrations of zero (a–c) and 10 mg/l (d–f). The magnification is 500x, 1000x, and 2000x, respectively.
Also, the pores on the surface of T. roseum became invisible because the external biomass surface had been covered by the superficial dye. The examination of SEM showed a high affinity of T. roseum for the Lanasyn Navy M-DNL dye, confirming the biosorption process; these results are in agreement with those of Danouche et al., who studied Mycoremediation of the Azo dye Acid Red 14 by Wickerhamomyces Anomalus and observed that there was a difference in cellular morphology between the control and AR14-loaded cells.
The yeast cells exhibited a regular morphology and a translucent outer layer outside the cell surface31 before the biosorption of AR14. However, the cell becomes smooth and exhibits blurry textures after dye biosorption.
The FTIR spectra were used to characterise the changes in functional groups of the residual dye culture media before and after inoculation by T. roseum. The FT-IR spectra are shown in Fig. 4. For the control spectrum, as shown in Fig. 4a, the absorption peaks were assigned at 3326.38 cm−1, which is attributed to hydroxyl groups; 2122.86 cm−1, which is attributed to allenes, ketenes, isocyanates, and isothiocyanates groups; 1635.54 cm−1, which is ascribed to the bend of the amide group; and 1055.92 cm−1, which is attributed to the sulfoxides group. Other absorbers with peaks at 411.10 cm−1, which are assigned the bromide and the iodide groups after the inoculation of Lanasyn Navy M-DNL dye with T. roseum, as shown in Fig. 4b, had peaks at 3326.38 cm−1, 2122.86 cm−1, 1635.54 cm−1, 1635.54 cm−1, 1055.92 cm−1 and 411.10 cm−1, which shifted to peaks at 3326.50 cm−1, 2103.68 cm−1, 1636.04 cm−1, 1045.30 cm−1 and at 419.31 cm−1, respectively.
Control (without T. roseum inoculation) (a) and after T. roseum inoculation (b).
The FT-IR spectra before and after residual dye media solution inoculation by T. roseum exhibited significant similarities in the spectra, which could be identified based on the reports in previous studies32. So, we can infer from this analysis that T. roseum cells decolourized Lanasyn Navy M-DNL dye by biosorption. Moreover, the FT-IR results indicated the main functional groups for biosorption of Lanasyn Navy M-DNL dye by T. roseum are hydroxyl, amide, and sulfoxides. On the contrary, Almeida & Corso observed alterations following Aspergillus terreus biodegradation treatment. They found that the FT-IR spectra of the binary solution exhibited significant changes after the biological treatment; this peak indicated complete breakage of the chemical bonds during biodegradation treatment; moreover, the breakage of these bonds led to the formation of aromatic amino compounds as intermediates19. Danoche et al. found that based on the comparison of the spectra before and after biosorption, a significant change was noticed in the transmittance of these functional groups, which may be attributed to the dye molecule31. A variety of functional groups, including amines, carboxyls, and phosphate groups, are present in the fungal cell walls and can bind dye molecules33. A terminal sodium sulphonate group, which can ionise into sodium cations and sulphonate anions, is what gives Lanasyn Navy M-DNL dye its distinctive properties. Therefore, sulphonate anions can be attracted to and removed from the fungus by its positively charged functional groups.
The results in Fig. 5a show that the optimum number of beads at which the maximum biosorption percentage of 94% of Lanasyn Navy M-DNL dye was achieved by immobilised T. roseum cells was 60 beads. It was shown that as the number of beads increased, the biosorption percentage decreased. The present investigation revealed the effect of bead numbers on the biosorption of Lanasyn Navy M-DNL dye. Also, it was found that 60 beads of immobilised T. roseum cells were the optimum number of beads at which the maximum biosorption of Lanasyn Navy M-DNL dye was achieved.
Bead numbers (a), fresh weight (b), temperature (c), pH (d), initial dye concentrations (e), and sucrose concentration (f), error bars are standard error of the mean.
The results in Fig. 5b show that the biosorption percentage increased with the increasing weight of T. roseum cells. Under the experimental conditions, the highest biosorption percentage of 97% was observed when using 1 g of fresh T. roseum cells.
Also, the results demonstrated that the biosorption percentage increased with the increasing weight of T. roseum cells. These results could be due to an attraction between dyes and biosorbents during the biosorption process.
The results in Fig. 5c show that the biosorption percentage of Lanasyn Navy M-DNL dye by immobilised cells of T. roseum was 96% at 25 °C, compared to other temperatures where the biosorption percentage was less than 96%; so 25 °C was chosen as the optimum temperature for biosorption of Lanasyn Navy M-DNL dye. Incubation temperatures exhibited variable rates of biosorption of Lanasyn Navy M-DNL dye.
Furthermore, it was discovered that 25 °C was chosen as the maximum temperature at which the highest biosorption percentage was obtained. Konicki et al. studied the effect of temperature on the equilibrium adsorption of AO8 and DR23 onto GO for three temperatures of 20 °C, 40 °C and 60 °C, at 10 mg l−1. They observed that the adsorption of AO8 decreased as the temperature increased, illuminating the exothermic nature of the adsorption mechanism. Contrarily, the adsorption equilibrium of DR23 increased as the temperature increased, showing that this dye attaches to GO through an endothermic process34.
The results in Fig. 5d show that pH 6 was the most effective in terms of the biosorption percentage of Lanasyn Navy M-DNL dye using T. roseum cells. pH 6, at which immobilised cells of T. roseum achieved a biosorption percentage of 96%.
Many authors have studied the effect of pH on dye decolourization, such as Mohamed et al., who observed that, as the pH increased, the removal per cent of red azo dye from aqueous solutions by Aspergillus niger increased and reached maximum removal efficiency (99.69%) at pH 9.0. By increasing the pH value, the decolourization decreased10. Also, Hema et al. found that the optimum decolourization of Azo acid orange-10 dye by Bacillus subtilis occurred at pH 8.535, and Shuhui et al. investigated the effect of pH value on the biosorption of dried-weight fungus pellets and showed that the colour change of three acid dyes decreased with the decrease in pH value.
That is to say, a lower pH helped with the adsorption of the acid anionic dyes36, since it was obvious that the colour was weaker when the dye solution was at a lower pH. Additionally, the biosorption of dyes is facilitated by changing the pH levels of dye solutions and adding protonated amino groups to the fungal biomass37.
Different concentrations of Lanasyn Navy M-DNL dye were tested to determine the optimum concentration, which was supported by the maximum biosorption percentage of Lanasyn Navy M-DNL dye by immobilised cells of T. roseum. The results in Fig. 5e show that the biosorption percentage reached 97% at a 10 mg/l Lanasyn Navy M-DNL dye concentration. Increasing the dye concentration resulted in a decrease in the biosorption percentage. Our findings support Neeta and Chattopadhyay’s assertion that at low initial dye concentrations, total removal of dye was observed because there were enough adsorption sites available for the dye molecules to bind. Reduced adsorption sites brought about a decrease in colour removal efficiency due to the rise in dye concentration38. Furthermore, Mohamed et al.‘s assertion that the decolourization process becomes slower as the original dye concentration is raised is also supported10. This could be a result of the adsorption sites on the adsorbent surface becoming less numerous.
The experiment aimed to study the sucrose concentration at which the highest biosorption percentage of Lanasyn Navy M-DNL dye could be achieved.
Results in Fig. 5f reveal that 10 g/l was the best sucrose concentration at which immobilised cells of T. roseum achieved a biosorption percentage of 96%. The sucrose concentration varies from one fungal species to another due to each fungal species having specific requirements for sucrose concentration for its growth and decolourization ability. Also observed was the rapid growth of the fungus in a C-limited medium with dye, which indicated that the fungus could utilise the dye as the sole source of carbon.
Langmuir and Freundlich’s adsorption isotherm models were used to determine the biosorption behaviour of immobilised T. roseum cells. The adsorption isotherm model for T. roseum is shown in Fig. 6a, b. The outcome revealed that when dye concentration increased, T. roseum’s dye adsorption capability appeared to trend upward before reaching equilibrium in a saturated state. Based on a study of the correlation coefficients (R2) of the two adsorption models (Langmuir and Freundlich adsorption isotherm models), Freundlich isotherms revealed high values of R2, suggesting that the adsorption process appeared to be a good fit for the Freundlich equation. This is explained by an increase in the interaction between adsorbate and mycelia, as previously reported by Abdolali et al.39. The Freundlich isotherm model implies heterogeneous sorption sites with various sorption energies. This model implies that the adsorption heat reduces exponentially with increasing coverage40 and is based on the non-uniform adsorption of the adsorbent surface.
Langmnir model (a) and Freunlich model (b).
The current study aims to investigate the possibility of reusing T. roseum living immobilised cells after elution with dist. H2O. The reuse of immobilised T. roseum cells for Lanasyn Navy M-DNL dye biosorption was tested by carrying out five successive elution cycles using distilled H2O as eluent. It is clear from the results in Fig. 7b that the ability of immobilised cells for biosorption of Lanasyn Navy M-DNL dye decreased from 95% in the first cycle to 80% in the fifth cycle so that the immobilised cells of T. roseum could be used several times. It could be due to the little destructive effect of the eluent on the immobilised cells of T. roseum. All applications that have been reported have demonstrated that the original bead shape was spherical. The repeated use, however, causes the alginate beads to degrade over time41.
Sixty beads were inoculated into a Lanasyn Navy M-DNL dye media flask (a). The ability of immobilised T. roseum cells for biosorption of Lanasyn Navy M-DNL dye, error bars are standard error of the mean (b).
Lanasyn Navy M-DNL dye, Isolan Black 2S-LDN dye, and Isolan Yellow 2S-GLN dye were provided by DyStar Colours Distribution, Heliopolis-Cairo, Egypt.
While Sodium alginate was purchased from Sigma Aldrich. Potato Dextrose Agar medium, Czapek’s Yeast Agar medium, and Dox’s medium were provided by a mycology laboratory, Department of Botany and Microbiology, Faculty of Science, Helwan University, Cairo, Egypt.
This study is to select a UPVC (unplasticised polyvinyl chloride) pipe manufacturing plant, Misr El-Nour Company, in the tenth of Ramadan City, El-Sharqiya governorate, Egypt, to obtain a successful decolourizing microbiota. This microbiota culture was obtained using the sedimentation or “settlement plates” technique, in which a Petri plate containing potato dextrose agar medium42 is exposed to the environment of UPVC for a predetermined amount of time (typically 15–60 min), then covered and incubated at 25 °C43,44.
In addition to T. roseum isolation, bacteria and yeast were obtained from an indoor UPVC factory. Firstly, the identification of T. roseum was made through microscopic examination and growth, according to Pitt and Hocking45 in the mycology Lab, Department of Botany and Microbiology, Faculty of Science, Helwan University, Cairo, Egypt. Then, using molecular identification46, the following was done to confirm the identification: growing cultures were delivered to the Molecular Biology Research Unit at Assiut University, where DNA was extracted using the Patho-gene-spin DNA/RNA extraction kit made available by the Korean company Intron Biotechnology. The rRNA gene and PCR (polymerase chain reaction) results on the fungal DNA examined in Daejeon, South Korea, by SolGent Company. The purified PCR result (amplicons) was sequenced with the incorporation of ddNTPs (Dideoxynucleotide triphosphate) in the reaction mixture46. The obtained sequences were analysed using a basic local alignment search tool from the NCBI website (National Centre for Biotechnology Information). A phylogenetic analysis of sequences was done with the help of MegAlign (DNA Star) software version 5.05.
This experiment was carried out in 250 ml flasks containing fifty ml of Dox′s medium47, to which the dyes Lanasyn Navy M-DNL, Isolan Black 2S-LDN, and Isolan Yellow 2S-GLN were separately added at an initial concentration of 10 mg/l, then inoculated with one mycelium disc (8 mm in diameter), and incubated for 7 days at 25 °C under static conditions. Flasks not inoculated with T. roseum were used as controls.
According to the decolourization assay, the percentage of decolourization was calculated. Three copies of each treatment were performed.
The optical density (absorbance) of the culture supernatant after centrifugation at 6000 rpm for 10 min (Hettich Zentrifugen Mikro 22RD-78532 Tuttlingen) was measured using a T60 UV–VIS Spectrophotometer to evaluate the degree of decolourization. Equation (1) was used to calculate the decolourization percentage according to48
Where A0 = absorbance at zero time without inoculation (control) (Fig. 2b), and AT = absorbance (after inoculation and incubation) (Fig. 2c). The maximum wavelength of the used dyes (Table 1) was determined at the central lab of the Faculty of Science, Helwan University, Cairo, Egypt, using UV/Vis spectrophotometry (Jasco-V-530).
Lanasyn Navy M-DNL dye has the highest decolourization percentage, according to the results of the experiment screening the decolourization percentage of the used dyes.
The surface morphologies of T. roseum in solution with Lanasyn Navy M-DNL dye concentrations of zero and 10 mg/l were monitored with a scanning electron microscope (SEM). Samples for SEM were prepared according to Ozturk et al.49.
After decolourization, the residual dye culture media (before and after inoculation by T. roseum) were analysed using FT-IR (Perkin Elmer Spectrum 10.5.4) in Cairo, Egypt. According to Chen et al.40, with a slight modification, the supernatant was collected by centrifugation, then evaporated and finally dried using an oven at 50 °C, followed by FT-IR analysis.
To optimise the biosorption of Lanasyn Navy M-DNL dye, it was first necessary to prepare the T. roseum cultures’ beads as previously described50. For this, an inoculum of T. roseum was taken from a seven-day Czapek’s yeast Agar51 slant culture, and 9 ml of sterile distilled water was added to each slant before being thoroughly shaken to create a T. roseum suspension. After shaking, 3 ml of the T. roseum suspension was inoculated into 50 ml of Dox’s broth medium in 250 ml flasks. The flasks were incubated on a shaker (150 rpm) for 48 h at 25 °C. After this period, T. roseum cells were harvested by filtration from the growth medium and washed several times with sterilised distilled water. Then, immobilisation of T. roseum cells via entrapment was prepared according to Arica et al.52 with appropriate modifications as follows: Na alginate (3.0 g was dissolved in 100 ml of distilled water) was used as a supporting material. The solution was sterilised at 121 °C for 15 min. After cooling to 40 °C, they were mixed with T. roseum cells (3% Na alginate, containing 0.25 g fresh weight).
The mixture was introduced drop by drop into a sterilised 2.5% anhydrous CaCl2 solution for 1 hour using a 3 ml syringe. The prepared beads’ diameter was determined using a micrometre, and the result was 4.4 mm. After being cured in this solution for two hours to ensure complete precipitation, the T. roseum-entrapped beads were rinsed twice with 200 ml of sterilised distilled water to get rid of extra calcium ions and unentrapped cells. The immobilised cells were then transferred to 50 ml of Dox’s broth medium. After the incubation at 25 °C for 24 h, the Ca-alginate beads with immobilised cells of T. roseum were removed from the growth medium by a tea strainer and rinsed twice with sterilised distilled water. The immobilised preparations were then kept at 40 °C in a 5 mmol/l CaCl2 solution until they were used for biosorption of Lanasyn Navy M-DNL dye.
Studying the impact of different parameters (beads number, fresh weight, temperature, pH, dye concentration, and sucrose concentration) on the biosorption of Lanasyn Navy M-DNL dye by immobilised cells of T. roseum.
In this experiment, every 250 ml flask was inoculated separately with various numbers of beads (20, 40, 60, and 80 beads) that contained fresh weights of T. roseum cells. After incubation, biosorption (%) was calculated.
The fresh weights of T. roseum (0.25, 0.5, 0.75, and 1 g) were mixed separately with 3% of the Na-alginate solution, followed by inoculation of each fresh weight into 50 ml of the dye media solution, which was then incubated to calculate the biosorption percentage of the Lanasyn Navy M-DNL dye.
Flasks were inoculated with 50 ml of Dox’s broth medium and 10 mg/l of the Lanasyn Navy M-DNL dye, which were then incubated for 7 days at temperatures of 20, 25, 30, 35, and 40 °C. The dye biosorption was then calculated.
Initial pH values of flasks containing the medium were set at various values (5, 6, 7, 8, 9, and 11), to which Lanasyn Navy M-DNL dye was added at a concentration of 10 mg/l. These flasks were also incubated for 7 days at 25 °C.
Initial dye concentrations investigated were 5, 10, 15, 20, and 25 mg/l. The flasks were inoculated, and at the end of the incubation period, the percentage of dye biosorption was calculated.
The medium was amended with different sucrose concentrations (0, 5, 10, 15, 20, 25, and 30 g/l). The biosorption percentage (Q%) was calculated after 7 days by the equations32
Where C0 and Ce are the starting concentration and final concentration of dye in the culture medium (mg l−1), qe is the adsorption capacity of the adsorbent (mg g−1) at the adsorption equilibrium, V is the broth volume (L), and m is the mass of the adsorbent (g).
Langmuir and Freundlich adsorption isotherm models were used to simulate Lanasyn Navy M-DNL dye adsorption by immobilised cells of T. roseum under optimised conditions of pH 6, a temperature of 25 °C, a concentration of 10 g l−1 fungal biomass, and the isothermal adsorption process of immobilised T. roseum cells at different Lanasyn Navy M-DNL dye concentrations (5–25 mg l−1). The Langmuir model (Eq. 4) and the Freundlich model (Eq. 5) were expressed as follows:
Where qe is the equilibrium adsorption capacity (mg g−1), Ce is Lanasyn Navy M-DNL dye concentration in the solution at equilibrium (mg L−1), KL is the Langmuir equilibrium parameter (L mg−1) and is related to the affinity at the binding site, and KF and n are Freundlich constants, representing the adsorption capacity and adsorption strength, respectively.
In this experiment, the elution cycle was repeated 5 times to reuse 60 beads of immobilised T. roseum cells (Fig. 7a). The beads were inoculated into Lanasyn Navy M-DNL dye media solutions, and after incubation at 25 °C for 7 days, the biosorption percentage was calculated after each elution cycle. Then, the beads were removed and gently washed with sterilised distilled water several times. After washing, those beads were re-suspended in flasks containing 50 ml of dye media solution and incubated at 25 °C for 7 days.
All data generated or analysed during this study are included in this published article.
Asad, S., Amoozegar, M., Pourbabaee, A., Sarbolouki, M. & Dastgheib, S. Decolorization of textile azo dyes by newly isolated halophilic and halotolerant bacteria. Bioresour. Technol. 98, 2082–2088 (2007).
Sen, S., Raut, S., Bandyopadhyay, P. & Raut, S. Fungal decolouration and degradation of azo dyes: a review. Fungal Biol. Rev. 30, 112–133 (2016).
Singh, P. & Shukla, P. Systems biology as an approach for deciphering microbial interactions. Brief. Funct. Genom. 14, 166–168 (2015).
Rawat, D., Sharma, R., Karmakar, S., Arora, L. & Mishra, V. Ecotoxic potential of a presumably non-toxic azo dye. Ecotoxicol. Environ. Saf. 148, 528–537 (2018).
Aksu, Z., Kilic, N., Ertugrul, V. & Donmez, G. Inhibitory effects of chromium (Vl) and remazol black on chromium (Vl) and dyestuff removals by Trametes versicolor. Enzym. Micro. Technol. 40, 1167–1174 (2007).
Saratale, R., Saratale, G., Chang, J. & Govindwar, S. Decolorization and biodegradation of textile dye navy blue HER by Trichosporon beigelii NCIM-3326. J. Hazard Mater. 166, 1421–1428 (2009).
Mugdha, A. & Usha, M. Enzymatic treatment of waste containing dyestuffs using different delivery systems. Sci. Rev. Chem. Commun. 2, 31–40 (2012).
Xu, M. et al. Shewanella sp. is a dye-decolorizing bacterium isolated from activated sludge of a waste-water treatment plant. Int. J. Syst. Evol. Microbiol. 55, 363–368 (2005).
Tan, L., Li, H., Ning, S. & Hao, J. Aerobic decolourization and degradation of acid orange G (AOG) by suspended growing cells and immobilized cells of a yeast strain Candida tropicalis TL-F1. Appl. Biochem. Biotechnol. 174, 1651–1667 (2014).
Mahmoud, M., Mostafa, M., Mohamed, S. & Mahmoud, N. Bioremediation of red azo dye from aqueous solutions by Aspergillus niger strain isolated from textile wastewater. J. Environ. Chem. Eng. 5, 547–554 (2017).
Mondal, P., Majumder, C. & Mohanty, B. Laboratory-based approaches for arsenic remediation from contaminated water: recent developments. J. Hazard Mater. 137, 464–479 (2006).
Hai, F., Yamamoto, K. & Fukushi, K. Hybrid treatment system for dye wastewater. Crit. Rev. Environ. Sci. Technol. 37, 315–377 (2007).
Ali, H. Biodegradation of synthetic dyes—a review. Water Air Soil Pollut. 213, 251–273 (2010).
Kurade, M. B., Waghmode, T. R., Kagalkar, A. N. & Govindwar, S. P. Decolorization of textile industry effluent containing disperse dye scarlet RR by a newly developed bacterial-yeast consortium BL-GG. Chem. Eng. J. 184, 33–41 (2012).
Khan, R., Bhawana, P. & Fulekar, M. Microbial decolourization and degradation of synthetic dyes: a review. Rev. Environ. Sci. Biotechnol. 12, 75–97 (2013).
Kurade, M. B., Waghmode, T. R., Khandare, R. V., Jeon, B. H. & Govindwar, S. P. Biodegradation and detoxication of textile dye disperse red 54 by Brevibacillus laterosporus and determination of its metabolic fate. J. Biosci. Bioeng. 121, 442–449 (2016).
Francine, I. et al. Occurrence and risk assessment of an azo dye—the case of disperse red.1. Chemosphere 156, 95–100 (2016).
Asses, N., Ayed, L., Hkiri, N. & Hamdi, M. Congo red decolourization and detoxification by Aspergillus niger: removal mechanisms and dye degradation pathway. Biomed. Res. Int. 7, 1–9 (2018).
Almeida, E. J. & Corso, C. R. Decolorization and removal of toxicity of textile azo dyes using fungal biomass pelletized. Int. J. Environ. Sci. Technol. 16, 1319–1328 (2019).
Bayramoglu, G., Celik, G., Yalcın, E., Yılmaz, M. & Arıca, M. Modification of surface properties of Lentinus sajor-cajun mycelia by physical and chemical methods: evaluation of their Cr6+ removal efficiencies from aqueous medium. J. Hazard Mater. B 119, 219–229 (2005).
Aneeta, S. & Balaprasad, A. Fungal biosorption as an alternative for the treatment of dyes in wastewater: a review (1) 10, 26-43. https://doi.org/10.1080/21622515.2020.1869322 (2021)
Abraham, T., Jamuna, R., Bansilal, C. & Ramakrishna, S. Continuous synthesis of glucoamylase by immobilized fungal mycelium of Aspergillus niger. Starch-Starke 43, 113–116 (1991).
Fiedurek, J. & Ilczuk, Z. Glucose oxidase biosynthesis using immobilized mycelium of Aspergillus niger. World J. Microbiol. Biotechnol. 7, 379–384 (1991).
Margaritis, A. & Merchant, F. Advances in ethanol production using immobilized cell systems. Crit. Rev. Biotechnol. 2, 339–393 (1984).
Vassilev, N. & Vassileva, M. Production of organic acids by immobilized filamentous fungi. Mycol. Res. 96, 563–570 (1992).
Katzbauer, B., Narodoslawsky, B. & Moser, A. Classification system for immobilization techniques. Bioprocess Eng. 12, 173–179 (1995).
Norton, S. & D’Amore, T. Physiological effects of yeast cell immobilization: applications for brewing. Enzym. Microb. Technol. 16, 365–375 (1994).
Rezaee, A., Godini, H. & Bakhtou, H. Microbial cellulose as support material for the immobilization of denitrifying bacteria. Environ. Eng. Manag. J. 7, 589–594 (2008).
Vucˇurovic´, V., Razmovski, R. & Rebic´, M. A corn stem as a biomaterial for Saccharomyces cerevisiae cells immobilization for ethanol production. Chem. Ind. Chem. Eng. Q. 14, 235–238 (2008).
Sandra, T. et al. A biosorption isotherm model for the removal of reactive azo dyes by inactivated mycelia of Cunninghamella elegans UCP542. Molecules 17, 452–462 (2012).
Danouche, M., El Arroussi, H., & El Ghachtouli, N. Mycoremediation of the azo dye Acid Red 14 by Wickerhamomyces anomalus: 2 Physicochemical characterization, equilibrium, and kinetics studies. https://doi.org/10.21203/rs.3.rs-891092/v1 (2021).
Gao, J., Zhang, Q., Su, K., Chen, R. & Peng, Y. Biosorption of acid yellow 17 from aqueous solution by non-living aerobic granular sludge. J. Hazard. Mater. 174, 215–225 (2010).
Aksu, Z., Tatli, A. & Tunç, Ö. A comparative adsorption/biosorption study of acid blue 161: effect of temperature on equilibrium and kinetic parameters. Chem. Eng. J. 142, 23–39 (2008).
Konicki, W., Aleksandrzak, M., Moszyński, D. & Mijowska, E. Adsorption of anionic azo-dyes from aqueous solutions onto graphene oxide: Equilibrium, kinetic and thermodynamic studies. J. Colloid Interface Sci. 496, 188–200 (2017).
Hema, G., Vyshali, V. & Tippeswamy, B. Bio-degradation of azo dye acid orange-10 by a new isolate of Bacillus subtilis isolated from soil sample around textile industry in South Karnataka. Biosci. Biotechnol. Res. Asia 17, 707–716 (2020).
Shuhui, L., et al. Mycoremediation of the Azo dye Acid Red 14 by Wickerhamomyces Anomalus: physicochemical characterization, equilibrium, and kinetics studies. R. Soc. Chem. J. https://doi.org/10.21203/rs.3.rs-891092/v1 (2021).
Fu, Y. & Virataghavan, T. Dye biosorption sites in Aspergillus niger. Bioresour. Technol. 82, 139–145 (2002).
Neeta, A. & Chattopadhyay, S. Biosorption of Azo dyes by spent Rhizopus arrhizus biomass. Appl. Water Sci. 7, 3041–3054 (2017).
Abdolali, A. et al. Characterization of a multi-metal binding biosorbent: chemical modification and desorption studies. Bioresour. Technol. 193, 477–487 (2015).
Chen, T. et al. Characterization and mechanism of copper biosorption by a highly copper-resistant fungal strain isolated from copper-polluted acidic orchard soil. Environ. Sci. Pollut. Res. 25, 24965–24974 (2018).
Damayanti, A., Kumoro, A., & Bahlawan, Z. Review calcium alginate beads as immobilizing matrix of functional cells: extrusion dripping method, characteristics, and application. International Conference on Chemical and Material Engineering (ICCME 2020) IOP Conf. Series: Materials Science and Engineering 1053 (2021). 012017IOP Publishing https://doi.org/10.1088/1757-899X/1053/1/012017.
Park, J. K. & Chang, H. N. Microencapsulation of microbial cells. Biotechnol. Adv. 18, 303–319 (2000).
Riker, A. J. & Riker, R. S. Introduction to research on plant diseases. John’s Swift Co. New York and Indianapolis. 117p. 1936.
Samson, R. A. & Frisvad, J. C. Penicillium sub-genus Penicillium: new taxonomic schemes, mycotoxins and other extra-litres. Studies in Mycology 49: 1–260. Buswell JA. Fungal degradation of chlorinated monoaromatics and BTEX compounds. In (ed Gadd G. M.). Fungi in Bioremediation. 113–135 (Cambridge: Cambridge University Press, 2004a).
Pitt, J. I. & Hocking, A. D. Fungi and food spoilage. Springer Nature Switzerland AG. 524 (2009).
White, T., Bruns, T., Lee, S. & Taylor, J. Amplification and direct sequencing of fungal ribosomal RNA genes for phylogenetics. In PCR Protocols: A guide to Methods and Applications (ed M. A. Innis, D. H. Gelfand, J. J. Sninsky & T. J. White). 315–322. (Academic Press, San Diego, USA, 1990).
Thom, G. & Raper, K. A Manual of the Aspergilla. Willams and Wilkins Co. Baltimore: 363 (1945).
Olukanni, O. D., Osuntoki, A. A. & Gbenle, G. O. Textile effluent biodegradation potentials of textile effluent adapted and non-adapted bacteria. Afr. Biotechnol. 5, 1980–1984 (2006).
Ozturk, S., Aslim, B. & Suludere, Z. Evaluation of chromium (VI) removal behaviour by two isolates of Synechocystis sp. In terms of exopolysaccharide (EPS) production and monomer composition. Bioresour. Technol. 100, 5588–5593 (2009).
Ilham, M. E., Azza, A. M. & Eman, H. A. Biosorption of pb and CD ions and decolourization wastewater of weaving and spinning textile factory by immobilized Pleurotus sajor-cajun and Pleurotus ostreatus. The First International Conference on Environmental Studies and Research. (pp. 494–509. Mnufiya University Sadat Branch, Egypt, 2008).
Samson, R. A. & Pitt, J. I. Advances in Penicillium and Aspergillus Systematics. 483 (Plenum Publishers, London and New York, 1985).
Arica, M., Arpa, C., Kaya, S., Denizli, A. & Genc, O. Comparative biosorption of mercuric ions from aquatic systems by immobilized life and heat-inactivated Trametes versicolor and Pleurotus sajor-cajun. Bioresour. Technol. 89, 145–154 (2002).
This paper is a self-funded research work, I am grateful to Dystar Colour Distribution for supplying their chemicals. Also, thank you to Misr El-Nour Company for supplying an isolated sample from their indoor UPVC pipe factory.
Open access funding provided by The Science, Technology & Innovation Funding Authority (STDF) in cooperation with The Egyptian Knowledge Bank (EKB).
Department of Botany and Microbiology, Faculty of Science, Helwan University, Cairo, 11795, Egypt
You can also search for this author in PubMed Google Scholar
The lone author of this paper and the corresponding author, is me, Dr. S.A.A. S.A.A. is the author of the methodology, validation, formal analysis, investigation, data curation, original draft, and review and editing of the writing. I reviewed the paper’s published form and approved it.
Correspondence to Sally A. Ali.
The author declares no competing interests.
Publisher’s note Springer Nature remains neutral with regard to jurisdictional claims in published maps and institutional affiliations.
Open Access This article is licensed under a Creative Commons Attribution 4.0 International License, which permits use, sharing, adaptation, distribution and reproduction in any medium or format, as long as you give appropriate credit to the original author(s) and the source, provide a link to the Creative Commons license, and indicate if changes were made. The images or other third party material in this article are included in the article’s Creative Commons license, unless indicated otherwise in a credit line to the material. If material is not included in the article’s Creative Commons license and your intended use is not permitted by statutory regulation or exceeds the permitted use, you will need to obtain permission directly from the copyright holder. To view a copy of this license, visit http://creativecommons.org/licenses/by/4.0/.
Ali, S.A. Decolourization of azo Lanasyn Navy M-DNL dye by Trichothecium roseum toward green mycoremediation. npj Clean Water 6, 64 (2023). https://doi.org/10.1038/s41545-023-00276-4
DOI: https://doi.org/10.1038/s41545-023-00276-4
Anyone you share the following link with will be able to read this content:
Sorry, a shareable link is not currently available for this article.
Provided by the Springer Nature SharedIt content-sharing initiative
npj Clean Water (npj Clean Water) ISSN 2059-7037 (online)
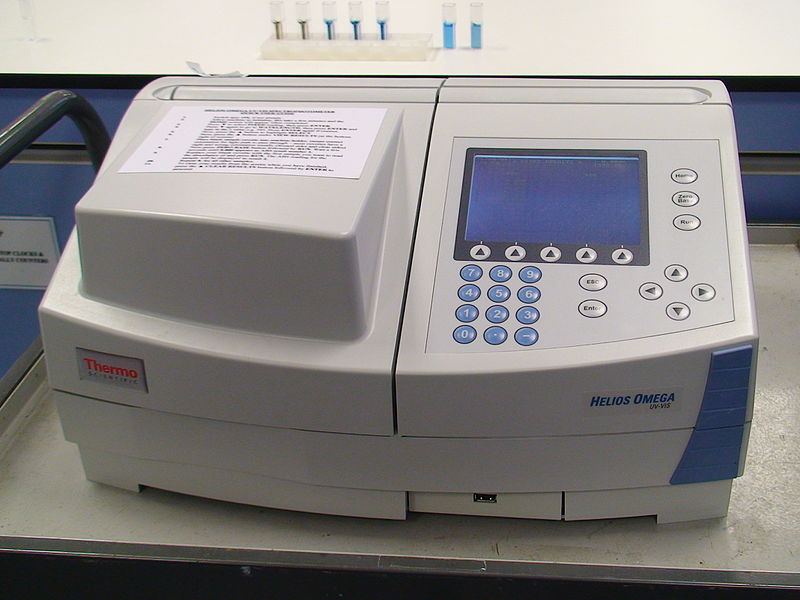
Perylene Red Sign up for the Nature Briefing newsletter — what matters in science, free to your inbox daily.