Thank you for visiting nature.com. You are using a browser version with limited support for CSS. To obtain the best experience, we recommend you use a more up to date browser (or turn off compatibility mode in Internet Explorer). In the meantime, to ensure continued support, we are displaying the site without styles and JavaScript.
Scientific Reports volume 12, Article number: 12004 (2022 ) Cite this article 2,4-Dihydroxy-6-methylpyrimidine
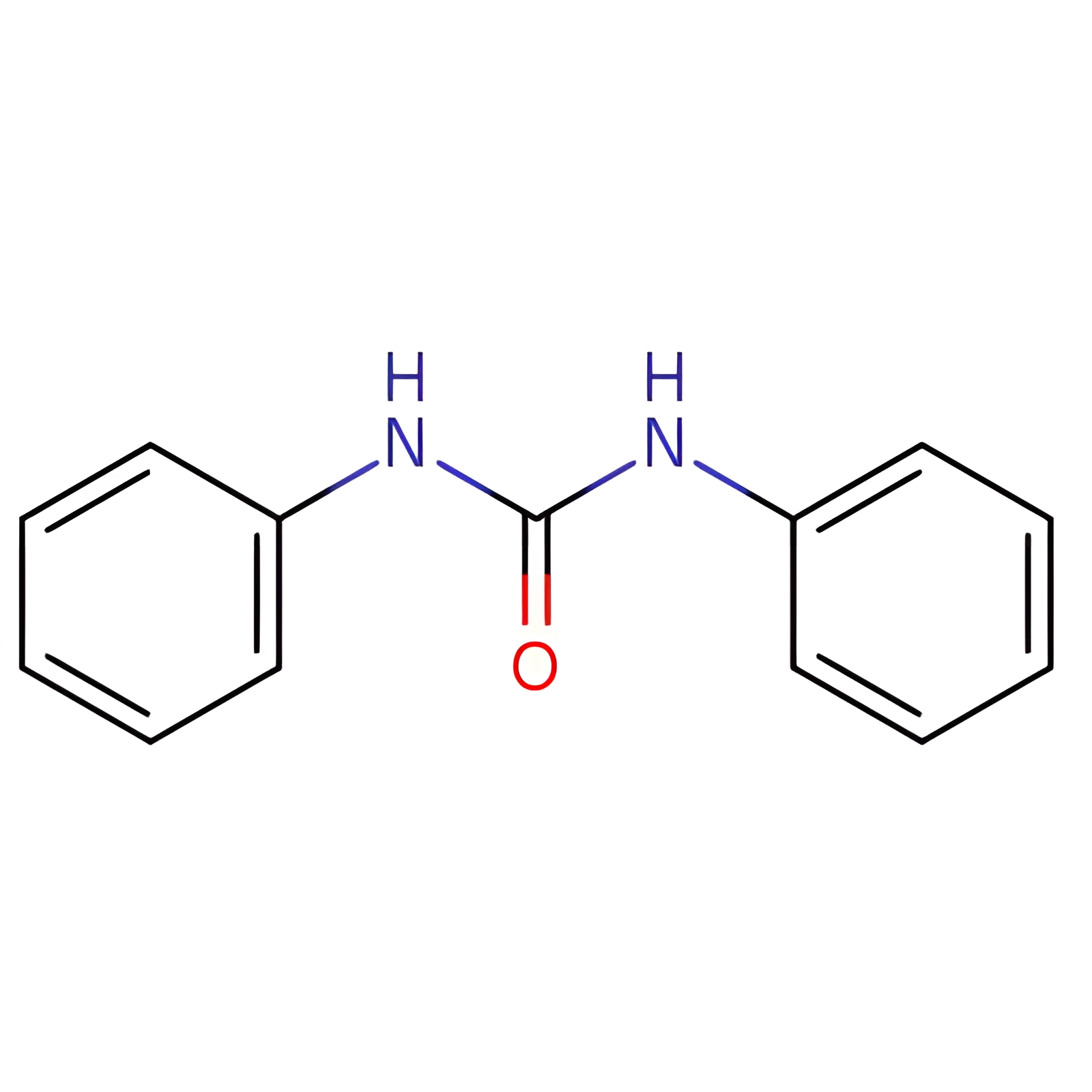
Lanthanoid-containing polyoxometalates (Ln-POMs) have been developed as effective and robust catalysts due to their Lewis acid–base active sites including the oxygen-enriched surfaces of POM and the unique 4f. electron configuration of Ln. As an extension of our interest in Ln-POMs, a series of as-synthesized nanocatalysts K15[Ln(BW11O39)2] (Ln-B2W22, Ln = La, Ce, Nd, Sm, Gd, and Er) synthesized and fully characterized using different techniques. The Ln3+ ion with a big ionic radius was chosen as the Lewis acid center which is sandwiched by two mono-lacunary Keggin [BW11O39]9− units to form Ln-containing sandwiched type cluster. Consequently, the catalytic activity of nanocatalysts with different Ln was examined in the synthesis of bioactive isatin derivatives and compared under the same optimized reaction conditions in terms of yields of obtained products, indicating the superiority of the nano-Gd-B2W22 in the aforementioned simple one-pot reaction. The effects of different dosages of nanocatalyst, type of solvent, reaction time, and reaction temperature in this catalytic system were investigated and the best results were obtained in the presence of 10 mol% of nano-Gd-B2W22 in water for 12 min at the reflux condition.
Xingxing Ma, Mengwei Tan, … Qiuling Song
Yuan-Xu Jiang, Li-Li Liao, … Da-Gang Yu
Pei-Ye You, Kai-Ming Mo, … Dan Li
The term “spiro” in organic chemistry was firstly defined by Von Baeyer in the late 1890s. This term is used when two hydrocarbon rings are assembled on a shared carbon atom which is named the spiro carbon atom. Presently, spiro organic structures are considered in designing new pharmaceuticals. The special biological and conformational characteristics with the complexity and rigidity properties of the spiro compounds, make them good chiral candidates in drug discovery1,2,3,4.
Spirooxindole core is one of the most popular spiro compounds found in the structure of many alkaloids, bioactive synthetic compounds, and pharmaceuticals (Fig. 1)5. Spirooxindoles have shown various biological activities, including promising anticancer6, antimicrobial7, antiviral, antioxidant, anti-inflammatory, antileishmanial, and antiplasmodial agents8,9. Moreover, some spirooxindole-based compounds have been developed as inhibitors of microtubule assembly, such as spirotryprostatin A, alstonisine, and ptropodind. According to the importance of spirooxindoles in drug discovery, many researches have been directed to find new efficient synthetic routes furnishing molecules containing this core10,11.
Polyoxometalates (POMs), known as inorganic ligands, are discrete, anionic metal-oxide clusters of group V or VI transition metals in their highest oxidation state and exhibit a great diversity of sizes, nuclearities, and shapes12,13,14. POMs benefit from interesting structural skeletons including protons (Brönsted acids, can promote acid‐catalyzed reactions), oxygen atoms (with a high negative charge can be used in base‐catalyzed reactions), and metal ions with unoccupied orbitals (Lewis acids)15. The motivation for choosing POMs comes not only from their intriguing structural diversity, but also they contain several potential applications in many fields such as sorbent16,17 catalysis18,19,20, magnetic21, optical materials sensitive devices22, electro/photochromic systems23, sensors24 and medicine25. Lacunary POMs are defect derivatives of saturated ones, including one or more vacant sites such as mono-lacunary, di, or tri-lacunary structures26. The most common lacunary POMs are derivatives of the Keggin and Wells–Dawson ions, resulting frequently in sandwich-type clusters27,28. Totally, structural vacancies in the lacunary POMs lead to enhance surface reactivity, therefore, they can be substituted by metals with strong Lewis acidity, such as lanthanoids or transition metals like zirconium to generate Lewis acid catalysts29,30.
Lanthanoid-containing polyoxometalates (Ln-POMs), specially constructed from lacunary Keggin anions are structurally rigid clusters (Fig. 2) and showed higher stability, and have Lewis acid–base active sites compared with naked POMs. Also, a synergistic combination between the Ln and POM within one molecular structure can enhance their potential application in many fields such as luminescence, magnetism, and catalysis31,32. Furthermore, due to their easy synthetic procedure and their robustness nature in the solid and solution, they can be also used in acid/base-catalyzed reactions for laboratory research purposes and industrial applications. Although there are several examples of isatin-based compounds synthesized using POMs or POMs-based composites33,34,35,36, Lewis acid catalysts containing Ln-POMs have rarely been studied for them.
Reproduced from ref40 with permission.
The structure of Ln-B2W22 nanocatalyst (Color code: W, dark blue; Ln, grey; O, red; B, purple).
Herein, we have successfully synthesized a series of isostructural α-Keggin borotungstate dimers with Ln cations, [Ln(BW11O39)2]15− (Ln-B2W22, Ln = La, Ce, Nd, Sm, Gd, and Er). Then, the related nanocatalysts were prepared by the top-down approach using the ultrasonic technique. In continuation of our efforts towards advancing synthetic methods to achieve spirooxindoles, in this research, we want to introduce a highly efficient, environmentally benign, and simple one-pot method for the nano-Gd-B2W22-catalyzed synthesis of bioactive spirooxindole derivatives37,38,39.
The chemical compounds were purchased from Merck (Darmstadt, Germany, www.merckmillipore.com) and Sigma-Aldrich (St. Louis, MO, USA, www.sigmaaldrich.com) and used with no crystallization or purification.
Electrothermal 9200 apparatus was employed to determine the melting point of products. Bruker Tensor 27 FT-IR spectrometer (400–4000 cm–1 region) was used to detect absorbance bands of organic products using a KBr disk containing the compounds. 1H NMR, 13C NMR spectra were recorded on a Bruker AQS 400-AVANCE spectrometer at 400 and 100 MHz, respectively, using TMS as an internal standard (DMSO solution). Also, the infrared spectra of catalysts were recorded in the range of 4000–400 cm–1 on a Thermo Nicolet/AVATAR 370 Elemental analysis (CHN) was performed using a Thermo Finnigan Flash EA 1112 microanalyzer. Metal content was measured by the Spectro Arcos ICP-OES spectrometer model 76004555 using in the range of 130–770 nm for ICP spectra. Powder X-ray diffraction (PXRD) data were collected on ASENWARE/AW-XDM300 X-ray powder diffractometer using Cu Kα (λ = 1.54184 Å) radiation at room temperature with the scan range 2θ = 3 to 40° and step size of 0.05° and step time of 1 s. The scanning electron microscope (SEM) analysis, EDS, and EDS mapping were recorded using LEO-1450 VP at an acceleration voltage of 10.00 kV and resolution of about 500 nm (Zeiss, Germany).
The mono-lacunary Keggin K9[BW11O39]·13H2O was synthesized according to a literature method and identified by FT-IR and elemental analysis41. Then, mono-lacunary Keggin can be stabilized by lanthanide centers in solution and in the solid-state to form sandwich-type polyoxometalates K15[Ln(BW11O39)2]·nH2O (Ln-B2W22, Ln = La, Ce, Nd, Sm, Gd, and Er)40,42.
General synthetic procedure for catalysts. A mixture of lanthanoid nitrate (0.085 mmol) and K9[BW11O39]·13H2O (0.155 mmol) in 20 mL of KCl (1 M) was stirred for 10 min in air and then the pH was adjusted to 5.0 by dropwise addition of 0.1 M KOH. The resulted mixture was stirred for a further 40 min at 50 °C. Pure crystals of the catalysts were obtained by slow evaporation of the solvent after several days.
Synthesis of nanocatalysts. The mixture solution of Ethanol (10 mL), water (15 mL), and Ln-B2W22 crystals (0.03 g) were subjected to ultrasonication (150 W). After 20 min, nanocatalysts were collected by the centrifuge and then washed with cold water (3 × 5 mL) under vacuum. FT-IR spectra (KBr pellet, cm−1) of nano-Ln-B2W22 were consistent with their spectra before doing the nano procedure (Fig. S2).
General procedure for the synthesis of spiro-2-amino-4H-pryans. A combination of 1,3-diketone, carbonyl compound (either isatin or acenaphtoquinone), α-cyano compound (either malononitrile or ethyl cyanoactetate), and Gd-B2W22 was stirred in water at ambient temperature until the complete formation of the product was traced by TLC (Fig. 3). Then, the crude product was filtered, washed with water and dissolved in hot ethanol for crystallization. Furthermore, all products were characterized and analyzed by melting points and FT-IR spectra, and the results were compared with those reported in the literature to prove the formation of target products.
Synthesis of spiro-2-amino-4H-pryans.
General procedure for the synthesis of uracil fused spirooxindoles. A combination of isatin, uracil derivative (either 1,3-dimethyl-6-aminouracil or 6-aminouracil), 1,3-diketone compounds, and Gd-B2W22 was stirred in refluxing water for 8–26 min (Fig. 4). Then, the mixture was filtered, washed well with water and dried at 80 °C. The product was recrystallized for further purification in hot ethanol. All products were characterized by melting point and the characterizations were compared with that of in literature.
Synthesis of pyrroloacridine derivatives. A mixture of isatin, aniline, dimedone and nanocatalyst was refluxed in water for an appropriate time (Fig. 5). By the completion of the reaction, the mixture was cooled down and filtered. Then the crude product was washed well with hot water, and finally crystallized in hot EtOH. The characterization data of products were compared with that published in the literature.
La-B2W22. Colorless needle-like crystals in 52.6% yield (based on W). Anal. Calcd. for H52O104LaK15B2W22: K, 9.01; W, 65.2; La, 2.13; B, 0.33; H, 0.81%. Found: K, 9.37; W, 63.66; La, 2.38; B, 0.31; H, 0.77%. FT-IR (KBr pellet, cm−1): 3451, 1616, 1254, 997, 948, 887, 832, 777, 521.
Ce-B2W22. Orange needle-like crystals in 55% yield (based on W). Anal. Calcd. for H58O107CeK15B2W22: K, 8.94; W, 61.62; Ce, 2.13; B, 0.33; H, 0.89%. Found: K, 9.21; W, 60.75; Ce, 2.09; B, 0.31; H, 0.92%. FT-IR (KBr pellet, cm−1): 3446, 1616, 1252, 996, 947, 887, 831, 777, 522.
Nd-B2W22. Light purple needle-like crystals in 49% yield (based on W). Anal. Calcd. for H50O103NdK15B2W22: K, 9.03; W, 62.27; Nd, 2.22; B, 0.33; H, 0.78%. Found: K, 9.32; W, 63.41; Nd, 2.18; B, 0.31; H, 0.81%. FT-IR (KBr pellet, cm−1): 3441, 1617, 1243, 996, 984, 885, 832, 777, 520.
Sm-B2W22. Colorless needle-like crystals in 62% yield (based on W). Anal. Calcd. for H50O103SmK15B2W22: K, 9.02; W, 62.21; Sm, 2.31; B, 0.33; H, 0.78%. Found: K, 9.06; W, 63.41; Sm, 2.21; B, 0.31; H, 0.76%. FT-IR (KBr pellet, cm−1): 3438, 2917, 1611, 1253, 1000, 494, 884, 831, 778, 519.
Gd-B2W22. Colorless needle-like crystals in 65% yield (based on W). Anal. Calcd. for H60O108GdK15B2W22: K, 8.89; W, 61.30; Gd, 2.38; B, 0.33; H, 0.92%. Found: K, 9.01; W, 61.45; Gd, 2.31; B, 0.31; H, 98%. FT-IR (KBr pellet, cm−1): 3471, 1611, 1253, 1000, 948, 883, 832, 799, 517.
Er-B2W22. Colorless needle-like crystals in 53% yield (based on W). Anal. Calcd. for H52O104ErK15B2W22: K, 8.97; W, 61.88; Er, 2.56; B, 0.33; H, 0.80%. Found: K, 9.03; W, 61.51; Er, 2.51; B, 0.32; H, 0.91%. FT-IR (KBr pellet, cm−1): 3428, 1621, 1258, 997, 948, 886, 835, 780, 522.
Firstly, six lanthanoid-containing polyoxometalate K15[Ln(BW11O39)2] (Ln-B2W22, Ln = La, Ce, Nd, Sm, Gd, and Er) crystals (microscopic size) of this study were obtained by reaction of the lanthanoid ion with the mono-lacunary Keggin [BW11O39]9− at pH 5 (Figs. 2 and S3). Next, the above crystals were solved and subjected to ultrasonication and then nanocatalysts were collected by the centrifuge (top-down approach). The distribution histograms reveal that the average particle size of catalysts is less than 100 nm upon 20 min of sonication (Fig. 6). Particle size distribution histogram of other nanocatalysts are given in the Supplementary Figs. S4–S8.
Particle size distribution histogram of nano-Gd-B2W22.
Also, the SEM showed that the dominant morphology for nanocatalysts is rod-like (Fig. 7). Furthermore, the presence of O, K, Gd, and W in the nanocatalysts is confirmed by the EDS spectrum (Fig. 8). SEM images of La-B2W22 and EDS spectra of other nanocatalysts are given in Supplementary Information (Figs. S9–S14).
SEM images of nano-Gd-B2W22.
EDS spectrum of nano-Gd-B2W22.
It is important to note that infrared spectroscopy is frequently employed technique for the characterization of POMs due to their characteristic metal–oxygen stretching vibrations that occur in the region between 400 and 1000 cm−1 which is known as the fingerprint region for the POMs. As shown in Figs. S1, S2, and Table 1, the overlaid IR spectra strongly suggest the same structural family for all crystalline and nano compounds. Also, the IR spectra of catalysts present a similar vibration pattern with the mono-lacunary Keggin [BW11O39]9−, confirming the presence of the [BW11O39]9− moiety in all compounds. Briefly, nano-Gd-B2W22 showed the absorption bands at 1610 and 3471 cm−1 which attributed to the water molecules. The band at around 1250 cm−1 is attributed for bending frequencies of O–B–O. Also, characteristic bands of the terminal oxygens νas(W–Ot) at 948 cm−1 showed a red shift in comparison with the naked [BW11O39]9− (995 cm−1) that indicated [BW11O39]9− anions coordinated to Ln3+ center (Fig. 9).
FTIR spectrum of nano-Gd-B2W22.
Also, the powder XRD pattern of the catalysts appears at around 9–10° for a 2θ value (similar to other mono-lacunary Keggin anions)43 (Supplementary Fig. S15).
Ensuing this research, the catalytic activity of nano-Ln-B2W22 catalysts was tested in the model three-component reaction of isatin, malononitrile, and dimedone. To achieve eco-friendly optimized conditions, various factors were investigated. Initially, acidic catalysts including SSA, p-TSA, H3PW12O40, Lanthanum nitrate, K9[BW11O39]·13H2O and ZnO were chosen to compare the results obtained by catalysts (Table 2). The Gd-B2W22 nanocatalyst was selected for further tests. Next, the effect of solvent was studied by running the model reaction in polar and non-polar solvents. Finally, the amount of catalyst was optimized to achieve the highest amount of product. The reaction was also repeated with no catalyst furnishing trace amount of product. That’s while in the presence of 10 mol% of nanocatalyst, the target product was obtained in 96%. Therefore, ensuring by the effect of a catalyst in this reaction, the generalization was accomplished in water, in the presence of 10 mol% nano-Gd-B2W22 at reflux condition. It is important to note that the Lewis acidity (Z/r3; Z = charge and r = ionic radius) of lanthanoids decreases with an increase in the ionic radii44. However, among the Ln-B2W22 (Ln = La, Ce, Nd, Sm, Gd, and Er) catalysts examined, Gd-B2W22 showed better catalytic performance because by reducing the size from Gd to Er, the Er center was sterically hindered by two BW11 ligands and its Lewis acid site is not well accessible.
The one-pot reaction of isatin, α-cyano compound (either malononitrile or ethylcyanoacetate), and 1,3-diketone (either ethyl acetoacetate, dimedone, or barbituric acid) or 3-methyl-1H-pyrazol-5(4H)-one/ 4-hydroxycoumarin or α-naphtol/β-naphtol) gave the favorite products. Notwithstanding, the effect of substituent on isatin ring, the yield of products was found in good to high. By employing acenaphthenequinone instead of isatin, the expected spiro-4H-pyrans were formed in good to high yields. The products obtained from ethylcyanoacetate need a longer reaction time than those obtained from malononitrile that possibly is due to the lower reactivity of ethylcyanoacetate (Table 3). All products were known and identified by comparing their melting points with authentic literature. Some selected NMR spectra are presented in supplementary file (Figs. S16–S47).
In Scheme 1, we propose a sensible mechanism for the preparation of spirooxindole derivatives. First, the Gd-B2W22 catalyst, as a Lewis acid, activates the carbonyl group of the isatin molecule, and then malononitrile, due to alpha-activated hydrogens, will have a nucleophilic attack on activated carbon, which produces intermediate 1. This intermediate creates intermediate 2 by elimination of water, and finally the corresponding product was synthesized by adding dimedone to this intermediate.
The reasonable mechanism for the synthesis of spirooxindole derivatives.
To confirm the wide effectiveness of nano-Gd-B2W22 as a catalyst, this was used in the reaction of isatin derivatives, 6-amino-1,3-dimethyl uracil, and 1,3-diketone (either dimedone, 1,3dimethyl barbituric acid, or barbituric acid). These reactions were successfully catalyzed by nano-Gd-B2W22 in refluxing water under optimized conditions furnishing spiro-products in good efficiency. The results are summarized in Table 4.
Next, the catalytic effect of nano-Gd-B2W22 was studied in the production of pyrroloacridine compounds through the one-pot reaction of isatin, aromatic amines, and dimedone. The generalization of this reaction was considered using different aromatic amine-bearing electron-donating and electron-withdrawing substituents. The expected pyrroloacridine derivatives were formed in wonderful yield within short reaction times as summarized in Table 5 (Fig. 10).
Heterogeneous Catalysts, play an important and effective role in industries and other applications in laboratory scale. Hence, recyclability of the catalyst to prevent waste generation is one of the most important factors in catalysis. Nevertheless, recoverability of nano-Gd-B2W22 was evaluated on the model reaction and it was recycled up to 6 runs by simple filtration with a gradual decrease in activity from 96 to 85% in the corresponding product (Fig. 10). In addition, to elucidate whether the recycling process can result in any change in the catalyst’s morphology and structure, the SEM image as well as FTIR spectra of the recycled nano-Gd-B2W22 catalyst were recorded (Fig. 11). These results support that the structure of the nano-Gd-B2W22 underwent several reactions was preserved, but some agglomeration is evident.
(a) SEM image of recycled nano-Gd-B2W22; (b) FTIR overlay of the fresh and recycled nano-Gd-B2W22.
In the present study, a series of isostructural lanthanoid-containing polyoxometalate nanocatalysts Ln-B2W22 (Ln = La, Ce, Nd, Sm, Gd, and Er) were synthesized and characterized using a suite of analytical techniques. Among these nanocatalysts, the gadolinium-containing POM (Gd-B2W22) showed remarkable catalytic performance for the synthesis of bioactive isatin derivatives including spiro-2-amino-4H-pryans, uracil fused spirooxindoles, and pyrroloacridine derivatives under the reflux condition in high yields and short reaction times (8–26 min). Also, further studies are underway in our laboratory to extend the application of these family nanocatalysts to other coupling reactions.
The raw/processed data that supports the findings of this study is available from the corresponding authors upon reasonable request.
Rios, R. Enantioselective methodologies for the synthesis of spiro compounds. Chem. Soc. Rev. 41, 1060–1074. https://doi.org/10.1039/C1CS15156H (2012).
Article CAS PubMed Google Scholar
Ding, A., Meazza, M., Guo, H., Yang, J. W. & Rios, R. New development in the enantioselective synthesis of spiro compounds. Chem. Soc. Rev. 47, 5946–5996. https://doi.org/10.1039/C6CS00825A (2018).
Article CAS PubMed Google Scholar
Baeyer, A. Systematics and nomenclature of bicyclic hydrocarbons.Ber.German.Chem. Society.33, 3771-3775.https://doi.org/10.1002/cber.190003303187 (1900).
Saraswat, P., Jeyabalan, G., Hassan, M. Z., Rahman, M. U. & Nyola, N. K. Review of synthesis and various biological activities of spiro heterocyclic compounds comprising oxindole and pyrrolidine moities. Synth. Commun. 46, 1643–1664. https://doi.org/10.1080/00397911.2016.1211704 (2016).
Khafagy, M. M., Abd El-Wahab, A. H., Eid, F. A. & El-Agrody, A. M. Synthesis of halogen derivatives of benzo[h]chromene and benzo[a]anthracene with promising antimicrobial activities. Farm. 57, 715–722. https://doi.org/10.1016/S0014-827X(02)01263-6 (2002).
Kornet, M. J. & Thio, A. P. Oxindole-3-spiropyrrolidines and -piperidines. Synthesis and local anesthetic activity. J. Med. Chem. 19, 892–898. https://doi.org/10.1021/jm00229a007 (1976).
Article CAS PubMed Google Scholar
Abdel-Rahman, A. H., Keshk, E. M., Hanna, M. A. & El-Bady, S. M. Synthesis and evaluation of some new spiro indoline-based heterocycles as potentially active antimicrobial agents. Bioorg. Med. Chem. 12, 2483–2488. https://doi.org/10.1016/j.bmc.2003.10.063 (2004).
Article CAS PubMed Google Scholar
Okita, T. & Isobe, M. Synthesis of the pentacyclic intermediate for dynemicin a and unusual formation of spiro-oxindole ring. Tetrahedron 50, 11143–11152. https://doi.org/10.1016/S0040-4020(01)89417-5 (1994).
Usui, T., Kondoh, M., Cui, C.-B., Mayumi, T. & Osada, H. Tryprostatin A, a specific and novel inhibitor of microtubule assembly. Biochem. J. 333, 543–548. https://doi.org/10.1042/bj3330543 (1998).
Article CAS PubMed PubMed Central Google Scholar
Paul, S. & Das, A. R. Dual role of the polymer supported catalyst PEG-OSO3H in aqueous reaction medium: Synthesis of highly substituted structurally diversified coumarin and uracil fused spirooxindoles. Tetrahedron Lett. 54, 1149–1154. https://doi.org/10.1016/j.tetlet.2012.12.079 (2013).
Maheshwar Rao, B. et al. Carbon–SO3H: A novel and recyclable solid acid catalyst for the synthesis of spiro[4H-pyran-3,3′-oxindoles]. Tetrahedron Lett. 54, 2466–2471. https://doi.org/10.1016/j.tetlet.2013.02.089 (2013).
Yang, P. & Kortz, U. Discovery and evolution of polyoxopalladates. Acc. Chem. Res. 51, 1599–1608. https://doi.org/10.1021/acs.accounts.8b00082 (2018).
Article CAS PubMed Google Scholar
Mirzaei, M., Eshtiagh-hosseini, H., Alipour, M. & Frontera, A. Recent developments in the crystal engineering of diverse coordination modes (0–12) for Keggin-type polyoxometalates in hybrid inorganic–organic architectures. Coord. Chem. Rev. 275, 1–18. https://doi.org/10.1016/j.ccr.2014.03.012 (2014).
Taleghani, S., Mirzaei, M., Eshtiagh-hosseini, H. & Frontera, A. Tuning the topology of hybrid inorganic–organic materials based on the study of flexible ligands and negative charge of polyoxometalates: A crystal engineering perspective. Coord. Chem. Rev. 309, 84–106. https://doi.org/10.1016/j.ccr.2015.10.004 (2016).
Herrmann, S., Ritchie, C. & Streb, C. Polyoxometalate–conductive polymer composites for energy conversion, energy storage and nanostructured sensors. Dalton Trans. 44, 7092–7104. https://doi.org/10.1039/C4DT03763D (2015).
Article CAS PubMed Google Scholar
Derakhshanrad, S., Mirzaei, M., Streb, C., Amiri, A. & Ritchie, C. Polyoxometalate-based frameworks as adsorbents for drug of abuse extraction from hair samples. Inorg. Chem. 60, 1472–1479. https://doi.org/10.1021/acs.inorgchem.0c02769 (2021).
Article CAS PubMed Google Scholar
Akbari, M., Mirzaei, M. & Amiri, A. Synergistic effect of lacunary polyoxotungstates and carbon nanotubes for extraction of organophosphorus pesticides. Microchem. J. 170, 106665. https://doi.org/10.1016/j.microc.2021.106665 (2021).
Arab Fashapoyeh, M. et al. Photochemical and electrochemical hydrogen evolution reactivity of lanthanide-functionalized polyoxotungstates. Chem. Commun. 54, 10427–10430. https://doi.org/10.1039/C8CC06334F (2018).
Heravi, M. M. et al. H 5 BW 12 O 40 as a green and efficient homogeneous but recyclable catalyst in the synthesis of 4 H -Pyrans via multicomponent reaction. Appl. Organomet. Chem. 32, e4479. https://doi.org/10.1002/aoc.4479 (2018).
Tamimi, M. et al. Ag 3 [PMo 12 O 40 ]: An efficient and green catalyst for the synthesis of highly functionalized pyran-annulated heterocycles via multicomponent reaction. Appl. Organomet. Chem. https://doi.org/10.1002/aoc.5043 (2019).
Babaei Zarch, M. et al. Single-molecule magnets within polyoxometalate-based frameworks. Dalton Trans. 50, 15047–15056. https://doi.org/10.1039/D1DT01708J (2021).
Article CAS PubMed Google Scholar
Coronado, E., Giménez-Saiz, C. & Gómez-García, CJ Recent advances in polyoxometalate-containing molecular conductors.Coord.Chem.rev.249, 1776–1796.https://doi.org/10.1016/j.ccr.2005.02.017 (2005).
Walsh, J. J., Bond, A. M., Forster, R. J. & Keyes, T. E. Hybrid polyoxometalate materials for photo(electro-) chemical applications. Coord. Chem. Rev. 306, 217–234. https://doi.org/10.1016/j.ccr.2015.06.016 (2016).
Zhou, C., Li, S., Zhu, W., Pang, H. & Ma, H. A sensor of a polyoxometalate and Au-Pd alloy for simultaneously detection of dopamine and ascorbic acid. Electrochim. Acta 113, 454–463. https://doi.org/10.1016/j.electacta.2013.09.109 (2013).
Arefian, M., Mirzaei, M., Eshtiagh-Hosseini, H. & Frontera, A. A survey of the different roles of polyoxometalates in their interaction with amino acids, peptides and proteins. Dalton Trans. 46, 6812–6829. https://doi.org/10.1039/C7DT00894E (2017).
Article CAS PubMed Google Scholar
Jia, Q., Cao, J., Duan, Y. & Hu, C. The solution chemistry and reactivity of lacunary Keggin silicotungstates monitored in real-time by a combination of mass spectrometry and electrochemistry. Dalton Trans. 44, 553–559. https://doi.org/10.1039/C4DT02723J (2015).
Article CAS PubMed Google Scholar
Patel, A., Narkhede, N., Singh, S. & Pathan, S. Keggin-type lacunary and transition metal substituted polyoxometalates as heterogeneous catalysts: A recent progress. Catal. Rev. 58, 337–370. https://doi.org/10.1080/01614940.2016.1171606 (2016).
Lu, Y. et al. New polyoxometalate compounds built up of lacunary Wells−Dawson anions and trivalent lanthanide cations. Inorg. Chem. 45, 2055–2060. https://doi.org/10.1021/ic0515857 (2006).
Article CAS PubMed Google Scholar
Yekke-Ghasemi, Z. et al. Fabrication of heterogeneous-based lacunary polyoxometalates as efficient catalysts for the multicomponent and clean synthesis of pyrazolopyranopyrimidines. Inorg. Chem. Commun. 140, 109456. https://doi.org/10.1016/j.inoche.2022.109456 (2022).
Nie, Y.-M., Li, S.-H., Lin, M.-Y. & Yan, J. A micro-environment tuning approach for enhancing the catalytic capabilities of lanthanide containing polyoxometalate in the cyanosilylation of ketones. Chem. Commun. 56, 3809–3812. https://doi.org/10.1039/D0CC01216E (2020).
Narkhede, N., Singh, S. & Patel, A. Recent progress on supported polyoxometalates for biodiesel synthesis via esterification and transesterification. Green Chem. 17, 89–107. https://doi.org/10.1039/C4GC01743A (2015).
Lotfian, N., Heravi, M. M., Mirzaei, M. & Daraie, M. Investigation of the uncommon basic properties of [Ln(W5O18)2]9– (Ln = La, Ce, Nd, Gd, Tb) by changing central lanthanoids in the syntheses of pyrazolopyranopyrimidines. J. Mol. Struct. 1199, 126953. https://doi.org/10.1016/j.molstruc.2019.126953 (2020).
Pradhan, S. & Mishra, B. G. CsxH3-xPW12O40 nanoparticles dispersed in the porous network of Zr-pillared α-zirconium phosphate as efficient heterogeneous catalyst for synthesis of spirooxindoles. Mol. Catal. 446, 58–71. https://doi.org/10.1016/j.mcat.2017.12.013 (2018).
Sadjadi, S., Heravi, M. M., Malmir, M. & Masoumi, B. HPA decorated Halloysite Nanoclay: An efficient catalyst for the green synthesis of Spirooxindole derivatives. Appl. Organomet. Chem. 32, e4113. https://doi.org/10.1002/aoc.4113 (2018).
Heravi, M. M., Momeni, T., Mirzaei, M., Zadsirjan, V. & Tahmasebi, M. An amino acid@isopolyoxometalate nanoparticles catalyst containing aspartic acid and octamolybdate for the synthesis of functionalized spirochromenes. Inorg. Nano Metal Chem. 51, 896–909. https://doi.org/10.1080/24701556.2020.1813172 (2021).
Nazish, M. et al. Magnetic Fe 3 O 4 nanoparticle-supported phosphotungstic acid as a recyclable catalyst for the Kabachnik-fields reaction of isatins, imines, and aldehydes under solvent-free conditions. ChemPlusChem https://doi.org/10.1002/cplu.201402191 (2014).
Daraie, M., Beheshtiha, Y. S. & Heravi, M. M. Synthesis of spirochromene derivatives catalyzed by Mn(bpyo)2/MCM-41 in water. Monatshefte Chem. Chem. Mon. 146, 191–198. https://doi.org/10.1007/s00706-014-1292-8 (2015).
Kavyani, S. & Baharfar, R. Design and characterization of Fe 3 O 4 /GO/Au-Ag nanocomposite as an efficient catalyst for the green synthesis of spirooxindole-dihydropyridines. Appl. Organomet. Chem. 34, e5560. https://doi.org/10.1002/aoc.5560 (2020).
Abdelmoniem, A. M., Hassaneen, H. M. E. & Abdelhamid, I. A. An efficient one-pot synthesis of novel spiro cyclic 2-oxindole derivatives of pyrimido[4,5- b ]quinoline, pyrido[2,3- d :6,5- d′ ]dipyrimidine and indeno[2′,1′:5,6]pyrido [2,3- d ]pyrimidine in water. J. Heterocycl. Chem. 53, 2084–2090. https://doi.org/10.1002/jhet.2480 (2016).
Mougharbel, A. S. et al. Lanthanide-containing 22-tungsto-2-germanates [Ln(GeW 11 O 39) 2 ] 13–: Synthesis, structure, and magnetic properties. Inorg. Chem. 59, 4340–4348. https://doi.org/10.1021/acs.inorgchem.9b03271 (2020).
Article CAS PubMed Google Scholar
Tézé, A., Michelon, M. & Hervé, G. Syntheses and structures of the tungstoborate anions. Inorg. Chem. 36, 505–509. https://doi.org/10.1021/ic961051t (1997).
Bassil, B. S., Dickman, M. H., von der Kammer, B. & Kortz, U. The monolanthanide-containing silicotungstates [Ln(β-SiW11 O39)2]13 - (Ln = La, Ce, Sm, Eu, Gd, Tb, Yb, Lu): A synthetic and structural investigation. Inorg. Chem. 46, 2452–2458. https://doi.org/10.1021/ic061737d (2007).
Article CAS PubMed Google Scholar
Arun, S., Kumar, V., Naz, A., Suhail, S. & Kumar, P. Journal of the Indian Chemical Society A comparative catalytic study using different metal ions by incorporating functionalized metallosalen into the lacunary position of Keggin polyoxometalate. J. Indian Chem. Soc. 98, 100118. https://doi.org/10.1016/j.jics.2021.100118 (2021).
Tsuruta, H., Imamoto, T. & Yamaguchi, K. Evaluation of the relative Lewis acidities of lanthanoid(III) compounds by tandem mass spectrometry. Chem. Commun. https://doi.org/10.1039/a905569j (1999).
Daraie, M., Lotfian, N., Heravi, M. M. & Mirzaei, M. Chemoselective synthesis of drug-like pyrrolo[2,3,4-kl]acridin-1-one using polyoxometalate@lanthanoid catalyst. React. Kinet. Mech. Catal. 129, 391–401. https://doi.org/10.1007/s11144-019-01709-3 (2020).
Kefayati, H., Narchin, F. & Rad-Moghadam, K. An unexpected multicomponent reaction leading to 2-arylpyrrolo[2,3,4-kl]acridin-1(2H)-ones. Tetrahedron Lett. 53, 4573–4575. https://doi.org/10.1016/j.tetlet.2012.06.070 (2012).
M.M. gratefully acknowledges financial support from the Ferdowsi University of Mashhad, the Iran Science Elites Federation (ISEF), Zeolite and Porous Materials Committee of Iranian Chemical Society and the Iran National Science Foundation (INSF). This work is supported by Iran Science Elites Federation Grant No. M/98208, M/99397, and M/400230. Also, M.M.H. acknowledges financially supported by Alzahra University, Tehran, Iran.
The following authors contributed equally: Vadjiheh Sadat Amiri and Bita Abdolahi Sanati.
Department of Chemistry, School of Sciences, Alzahra University, Vanak, Tehran, Iran
Mansoureh Daraie & Majid M. Heravi
Department of Chemistry, Faculty of Science, Ferdowsi University of Mashhad, 9177948974, Mashhad, Iran
Masoud Mirzaei, Maryam Bazargan, Vadjiheh Sadat Amiri & Bita Abdolahi Sanati
You can also search for this author in PubMed Google Scholar
You can also search for this author in PubMed Google Scholar
You can also search for this author in PubMed Google Scholar
You can also search for this author in PubMed Google Scholar
You can also search for this author in PubMed Google Scholar
You can also search for this author in PubMed Google Scholar
M.D.: Methodology, Formal analysis, Investigation, Software, Data curation, Writing-original draft preparation. M.M.: Conceptualization, Funding acquisition, Supervision, Main idea, Writing-review and editing, Project administration, Visualization. M.B.: Methodology, Formal analysis, Investigation, Software, Data curation, Writing-original draft preparation. V.S.A.: Experimental work. B.A.S.: Experimental work. M.M.H.: Conceptualization, Funding acquisition, Supervision, Main idea, Writing-review and editing, Project administration, Visualization.
Correspondence to Masoud Mirzaei or Majid M. Heravi.
The authors declare no competing interests.
Springer Nature remains neutral with regard to jurisdictional claims in published maps and institutional affiliations.
Open Access This article is licensed under a Creative Commons Attribution 4.0 International License, which permits use, sharing, adaptation, distribution and reproduction in any medium or format, as long as you give appropriate credit to the original author(s) and the source, provide a link to the Creative Commons licence, and indicate if changes were made. The images or other third party material in this article are included in the article's Creative Commons licence, unless indicated otherwise in a credit line to the material. If material is not included in the article's Creative Commons licence and your intended use is not permitted by statutory regulation or exceeds the permitted use, you will need to obtain permission directly from the copyright holder. To view a copy of this licence, visit http://creativecommons.org/licenses/by/4.0/.
Daraie, M., Mirzaei, M., Bazargan, M. et al. Lanthanoid-containing polyoxometalate nanocatalysts in the synthesis of bioactive isatin-based compounds. Sci Rep 12, 12004 (2022). https://doi.org/10.1038/s41598-022-16384-z
DOI: https://doi.org/10.1038/s41598-022-16384-z
Anyone you share the following link with will be able to read this content:
Sorry, a shareable link is not currently available for this article.
Provided by the Springer Nature SharedIt content-sharing initiative
Journal of the Iranian Chemical Society (2023)
Journal of the Iranian Chemical Society (2023)
Journal of the Iranian Chemical Society (2023)
Journal of the Iranian Chemical Society (2023)
By submitting a comment you agree to abide by our Terms and Community Guidelines. If you find something abusive or that does not comply with our terms or guidelines please flag it as inappropriate.
Scientific Reports (Sci Rep) ISSN 2045-2322 (online)

Sodium 3-Chloro-2-hydroxypropanesulfonate Sign up for the Nature Briefing newsletter — what matters in science, free to your inbox daily.